Editor’s note:
This is the second in a trilogy of posts on Nick Lane’s theories of life and how they relate to the Fermi Paradox — or why we haven’t detected alien life yet. The first looked at how life first began. This post will look at how simple prokaryotes (bacteria and archaea) gave rise to complex eukaryotic life (animals, plants, fungi, and protists), and why it took so long.
Geochemistry first gave rise to biochemistry within alkaline hydrothermal vents, some 4 billion years ago. Inside the microscopic pores of those ancient rock formations, the first living organisms emerged from complex organic synthesis, and gave rise to LUCA — the last common ancestor of all life on Earth. These simple cells were fragile and unable to leave their home in the tranquil vent systems. To colonise the outer world, which at this time was almost entirely covered in ocean, life bifurcated into two branches.
Archaea and bacteria, the first two of the three domains of life, look very similar but are in fact incredibly distinct, biochemically-speaking. In evolutionary terms, there were two routes out of the vents; archaea went one way and bacteria the other. For the next few billion years, they evolved a dizzying diversity of genes and metabolic processes (essentially a chain of chemical reactions to release energy and grow), including many types of respiration and photosynthesis (which oxygenated the atmosphere for the first time). And yet, structurally-speaking, they’ve barely changed at all in four billion years. They’ve never evolved any complexity. How can that be?
Darwin’s theory of natural selection tells us that evolutionary change happens gradually over the course of many generations. Given how quickly bacteria and archaea replicate, they’ve had more than enough time for large-scale change to occur. Time was clearly not the limiting factor. Something else was holding them back.
Nick Lane’s thesis is that this constraint was energy. But before we can get into that, we need first to understand the differences between prokaryotic and eukaryotic cells.
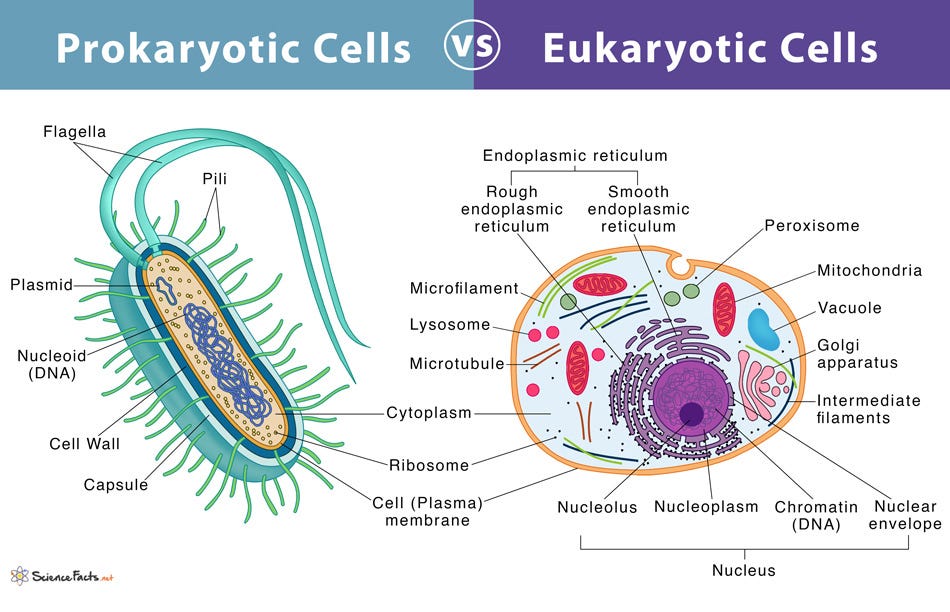
Just a passing glance at a cell diagram shows us the huge gulf in complexity between the first two and third domains of life. Bacteria and archaea just don’t have a lot of morphology to talk about; they don’t have many physical features. A loop of DNA lies within a cytoplasmic jelly, along with some ribosomes and a plasmid or two (smaller loops of DNA), which is all surrounded by a membrane. Some might have a flagellum, maybe some hairlike arms wafting around, or a vacuole, but otherwise there’s not a huge amount of structural variety. They’re simple little cells living a simple little life, never once in four billion years showing any inclination to something as innovative as multicellularity.
Meanwhile, all four kingdoms of eukaryotes show startling complexity in their cell structure: almost all eukaryotes have a nucleus, containing the organism’s DNA in sophisticated chromosomal formations; incredible network structures called endoplasmic reticula; mitochondria, the energy powerhouse organelle; a protein and lipid factory called a Golgi apparatus; while some also have other organelles like chloroplasts, which do photosynthesis in plants.
They differ in other ways too. Eukaryotic cells are much larger than prokaryotic cells, sometimes by as much as 100x, and have on average about 500x as many genes. However, perhaps the most interesting fact is that eukaryotes consume up to 200,000x more energy per gene. Cells tend to use about 80% of their energy to synthesise new proteins — to grow, essentially. Clearly the earliest eukaryotic cells were able to unlock some new means of energy production in order to grow so large and complex. But what was it?
A clue may lie in the startling similarity between all eukaryotic cells. Despite the cornucopia of biodiversity we see across plants, animals, fungi, and protists, their cells are remarkably similar. In fact, look at an animal cell and a fungi cell under a microscope and it can be hard to tell the difference. A mushroom isn’t much like an elephant, so why do their cells look the same? The complex organelles in eukaryotes must have evolved very early on after their evolutionary split from prokaryotes.
Life was stuck in simplicity for 2 billion years. Then something seismic happened. This event, whatever it was, must have unlocked the energy constraint that had restricted prokaryotes, and unleashed a torrent of evolutionary potential, changing cell size, structure, and, eventually, made possible the dizzying diversity of life that we now know. But what was it?